Their Importance, Usage and Applications to Medical Fields
Abstract
A sophomore college student performed this study on Nanoelectromechanical Systems (NEMS) in a project-based program. Topics discussed in this paper are the importance, usage and applications of the NEMS. The first author researched this technology and presented a poster at the ASEE annual conference; as a result, he is interested in further understanding the field of nanoelectromechanical engineering and its related issues and is also potentially inspired to pursue graduate studies in this area. Microelectromechanical systems (MEMS) use technology on the microscopic scale; the same can be said for NEMS on the nanoscale. NEMS can effectively collect data on the atomic level. Lightweight and low power requirements add to the versatility of these systems, which are being incorporated in a wide range of fields. The applications for these technologies go across multiple disciplines, including the medical field. Taking advantage of technologies at the nanoscale has already improved various forms of medical operations and instruments.
Introduction
The medical technology that has come to fruition due to the advances in NEMS technology has provided more options for treating patients, but nanomedicine has more it can offer. The United Nations Millennium Projects Task Force on Science, Technology and Innovations acknowledged the potential benefits of this technology by stating that nanotechnology has a lot to offer in disease and primary health care.1 NEMS technology can help treat diseases and infections, but its application can expand wider by improving diagnostics, wound treatment, and invasive operations such as surgeries. Today’s use of nanotherapeutics and nanodiagnostics shows what can be accomplished with this technology. Although NEMS are being used in the health industry, there are issues that researchers are attempting to solve in order to expand the use cases of this technology.
Background and body
A majority of the research done with nanomedicine is aimed at cancer treatment to provide better options to treat cancer as opposed to chemo and radiation therapy. These cancer treatment options are not only confined to one area of the body and have side effects but are also not effective at treating cancer that has spread to other areas of the body. There are already alternative solutions such as nanomedicine applications in breast cancer treatment that have already been produced and used. For example, current state-of-the-art “[n]anomedicine products such as Doxil® and Abraxane® have already been extensively used for breast cancer adjuvant therapy with favorable clinical outcomes.”2 But researchers are still looking for more effective cancer treatments. One researched concept is to take externally and internally modified c60 molecules that can hold cancer treatments inside, detect cancerous cells and deliver the treatment more accurately without killing healthy cells.
Adding to the areas of the medical field that NEMS can improve, instruments for diagnosing patients are an important tool for medical professionals. More accurate information from these instruments gives medical professionals a better understanding of the underlying issue. Scans, such as CT and PET scans, give medical professionals the information they need to care for patients. Combining these technologies results in a more accurate and informative scan than either scan by itself because having both images simultaneously and comparing the information from both scans quickly and effectively gives more information. The multicomponent capabilities of nanoparticles enable the culmination of these technologies resulting in the use of multimodality imaging in nanomedicine and nanotheranostics.3 Nanoparticles used in imaging help with scan visualization; similarly, these nanoparticles can be used to overcome the visibility issues related to dyes being used in surgeries. A variety of dyes have been used to assist in surgical operations. Dyes “such as fluorescein, indocyanine green, bromophenol blue, and Coomassie Blue [have] been attempted in brain tumor surgery.”2-8 Dyes have downsides that might outweigh their usefulness, such as difficulty in target differentiation, the need for special lighting conditions and short duration. Nanomaterials solve these issues using fluorescent nanoparticles. A research paper explains how nanoparticles can be used in this way: “conjugated iron oxide-based nanoparticles with near-infrared fluorescent dye Cy5.5 to form fluorescent nanoparticles as a new dye.”7 Due to the near-infrared that is nanoparticles give off, utilizing fluorescent nanoparticles requires a separate monitor to view the color differentiation. Another aspect of nanotechnology in the medical field is improved methods for invasive operations, most notably surgeries, as the following image shows.2
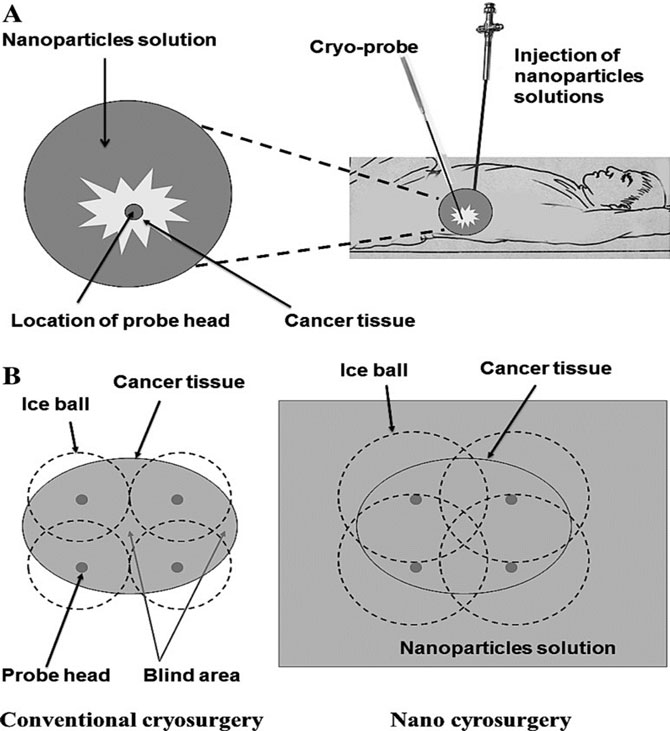
The comparison between conventional surgical methods and nanosurgery reveals notable differences. As shown in image 1, nanosurgery covers more of the cancer tissue, is less invasive than conventional surgery and takes up less area. The research paper’s description of this image shows the process of this method very well, “a. Specific nanoparticle solution is injected around cancer tissue to improve thermal conductivity, followed by insertion of cryo-probe under image guidance. b. Super-conductive nanoparticles lower the freezing temperature and the freezing zone (dashed line) around cancer tissue when compared to conventional cryosurgery.”2
Advances in surgical equipment, whether in the instruments themselves or tools that assist in the operation, are big contributions. There have also been exciting advances in the less invasive areas of medicine. NEMs have helped bring regenerative medicine closer to commercial use. A research paper defines this subject and its goals as follows: “the study of [the] application of nanomaterials or devices on damaged tissues or organs, aiming to promote tissue regeneration and repair with minimal scar and maximal function after injury.”2
As shown in image 2,8 three directions utilize nanotechnology, whether in conjunction with each other or separately, to achieve cell regeneration. Practical applications for this method are stated in a research paper that says, “In tissue engineering applications, magnetic force has been used to successfully manipulate iron oxide nanoparticles labeled cells in culture to create 3D tissue arrangements, including 3D small-diameter vascular grafts with three layers of cells containing iron oxide nanoparticles (endothelial, smooth muscle, and fibroblast cell layers).”9 These three methods, shown above, help heal various kinds of tissue ranging from bone to skin. Cells require various forms of support to live; conventional tissue engineering methods encounter issues with supplying these needs. With the advantage of nanonetworks and computational modeling, using scaffolds provides passageways for oxygen delivery to the tissue. The passageways are similar in structure to natural blood vessels.10
One of the most damaged organs in our bodies is our skin. The current process with wound treatment is to prevent infection and provide support in the body’s natural process of healing that wound. However, there are more sophisticated forms of treatment for complex wounds. “[S]tem cell therapy, gene therapy, photothermal and photodynamic therapy, [sic] are playing an increasingly vital role in some complicated wound treatment.”11 Nanotech solutions for wound treatment are still currently in development. Researchers are working on a nanodrug delivery system to improve the healing process. Image 3 shows the components of that system.11
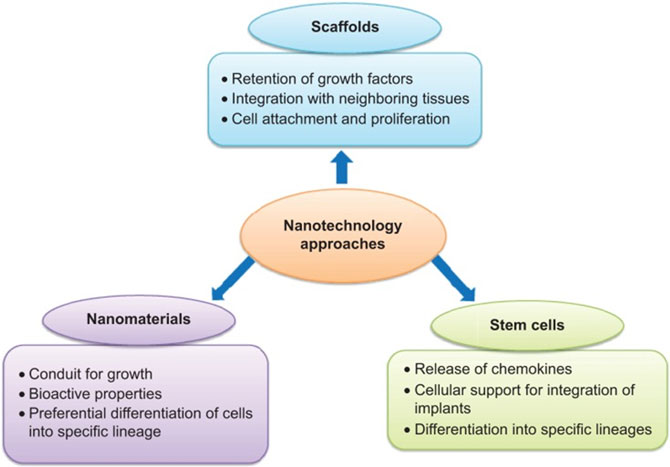
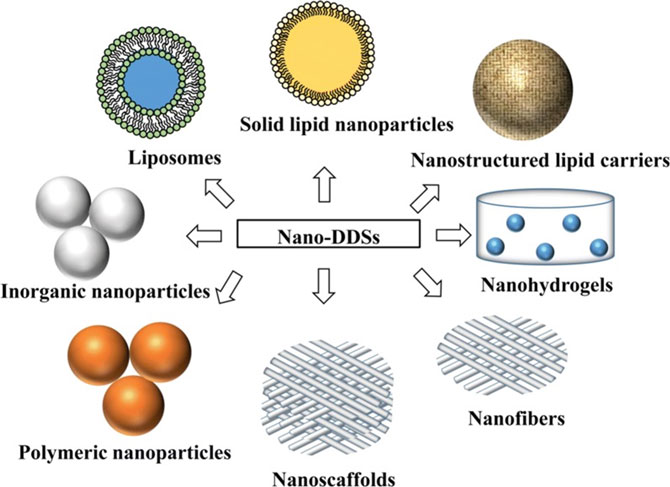
Liposomes serve as a carrier for treatment. They are hydrophobic and provide some resistance to being absorbed by the body and water. Polymeric nanoparticles protect the traveling drugs from degradation and help in the controlled release of the drugs. Inorganic nanoparticles such as silver are used as antimicrobial agents. Lipid nanoparticles also contribute to the controlled release of medication. As mentioned previously, nanofibrous structures or scaffolds allow proper nutrition to reach cells. Finally, the nanohydrogel provides fluid for the repair area so that dehydration doesn’t take effect. The culmination of all these parts of the nanodrug delivery system could improve tremendously how standard procedures are carried out.
Conclusion
NEMS applications in the health field are promising, whether in equipment, treatment, or other forms. The tip of the iceberg is being used for this technology; future research will bring far more options more safely and effectively. The current results will open doors for us in the future. The first author’s involvement in this project-based program interested him in the field of NEMS and related engineering fields and issues. He now sees this research as inspiration to potentially pursue graduate studies in this area. This project-based program has motivated the student and promoted the student’s interest to do further research in this area and possibly pursue a graduate degree in civil engineering or mechanical engineering at a research institution or university in the state. This research experience has been an educational benefit and outcome of this project-based program.
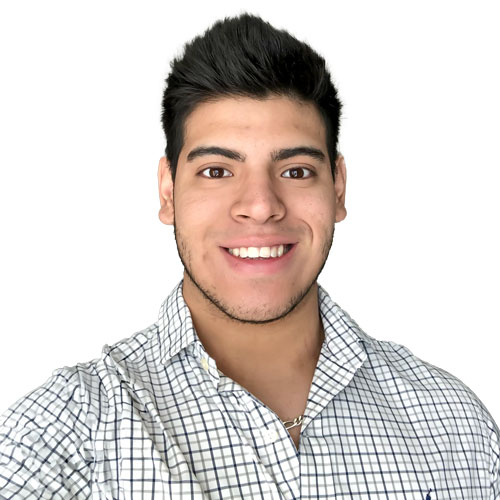
Ever since Sean Bonilla was young, his passion has been taking things apart, learning how they work, and improving what he took apart. He is majoring in electrical engineering at Salt Lake Community, where a nanotech class inspired him to research the benefits of nanotechnology in health care. His previous experience in computer programming, 3D printing and electronics gives him an interdisciplinary point of view on research and applications of nanotechnology to improve health care. His other experiences relating to STEM include completing the NASA Community College Aerospace Scholars Program and presenting a paper at UCUR 2022.
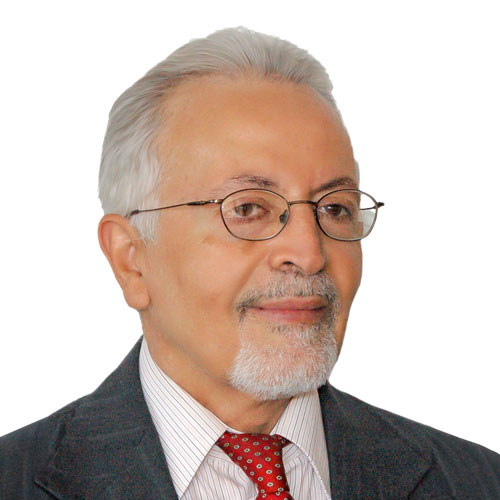
Dr. Nick Safai has four master’s degrees (in aerospace engineering, civil engineering, operation research and mechanical engineering), a Ph.D. degree in engineering and a one-year postdoctoral, all at Princeton University. He received his bachelor’s degree in mechanical engineering with a minor in mathematics from Michigan State. He has an extensive list of academic, industry and consulting accomplishments, including being an engineering department chair and professor since 1986. Nick is also an ASEE Fellow and the division chair of the ASEE International Division, and he has been an ASCE and ASEE officer and member for more than 30 years. He has won many awards for his service in various areas, including receiving recognition for leadership, teaching and writing. Nick has had a major role in establishing and developing the engineering department at Salt Lake Community College and expanding the ASEE International Division. During his term as the program chair, sessions increased from a few technical sessions to more than 20 sessions.
References and Citations
- Jillian Gardner. “Nanotechnology in Medicine and Healthcare: Possibilities Progress and Problems.” South African Journal of Bioethics and Law, no. 2, 2015, p. 50. EBSCOhost, doi:10.7196/SAJBL.432.
- 10. Wong KK, Liu XL. Nanomedicine: a primer for surgeons. Pediatric Surg Int 2012;28(10):943–951. Epub 2012 Aug 15. Review. PubMed PMID: 22892910.
See https://www.ncbi.nlm.nih.gov/pmc/articles/PMC3445794/ and https://pubmed.ncbi.nlm.nih.gov/22892910/. - Shinoda J, Yano H, Yoshimura S, et al. Fluorescence-guided resection of glioblastoma multiforme by using high-dose fluorescein sodium. Technical note. J Neurosurgeon. 2003; 99:597–603. See https://pubmed.ncbi.nlm.nih.gov/12959452/ and Google Scholar.
- Britz GW, Ghatan S, Spence AM, et al. Intracarotid RMP-7 enhanced indocyanine green staining of tumors in a rat glioma model. J Neurocoele. 2002; 56:227–232. See https://pubmed.ncbi.nlm.nih.gov/12061728/ and Google Scholar.
- Ozawa T, Britz GW, Kinder DH, et al. Bromophenol blue staining of tumors in a rat glioma model. Neurosurgery. 2005; 57:1041-1047. See https://pubmed.ncbi.nlm.nih.gov/16284574/ and Google Scholar.
- Orringer DA, Chen T, Huang DL, et al. The brain tumor window model: a combined cranial window and implanted glioma model for evaluating intraoperative contrast agents. Neurosurgery. 2010; 66:736-743. See https://www.ncbi.nlm.nih.gov/pmc/articles/PMC3970731/,
https://pubmed.ncbi.nlm.nih.gov/20305495/ and Google Scholar. - Veiseh O, Sun C, Fang C, et al. Specific targeting of brain tumors with an optical/magnetic resonance imaging nanoprobe across the blood-brain barrier. Cancer Res. 2009; 69:6200-6207,
https://pubmed.ncbi.nlm.nih.gov/19638572/ and Google Scholar. - Chaudhury K, Kumar V, Kandasamy J, Roy Choudhury S. Regenerative nanomedicine: current perspectives and future directions. Int J Nano med. 2014; 9:4153-4167. doi: 10.2147/IJN.S45332.
https://www.ncbi.nlm.nih.gov/pmc/articles/PMC4159316/,
https://pubmed.ncbi.nlm.nih.gov/25214780/,
https://www.dovepress.com/regenerative-nanomedicine-current-perspectives-and-future-directions-peer-reviewed-fulltext-article-IJN and Google Scholar. - Mclaughlin S, Podrebarac J, Ruel M, Suuronen E, McNeill B, Alarcon E. Nano-Engineered Biomaterials for Tissue Regeneration: What Has Been Achieved So Far? Frontiers in Materials 2016/06/27 10.3389/fmats.2016.00027.
- Wong K. Nanotech for New Organs. Scientific America 2003/07/09. See https://www.scientificamerican.com/article/nanotech-for-new-organs/.
- Wang, Wei et al. “Nano-drug delivery systems in wound treatment and skin regeneration.” Journal of nanobiotechnology vol. 17,1 82. 10 Jul. 2019, doi:10.1186/s12951-019-0514-y.